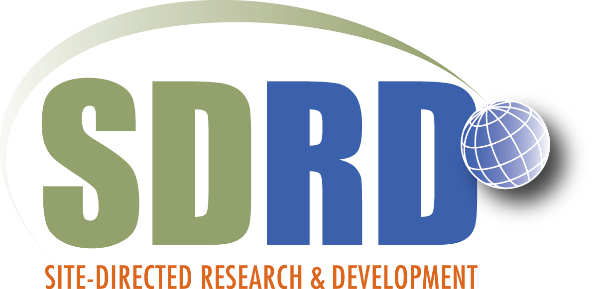
Refinements to the Geological Framework Models by Incorporating Multiscale Variability Constrained by Seismic Array Observations
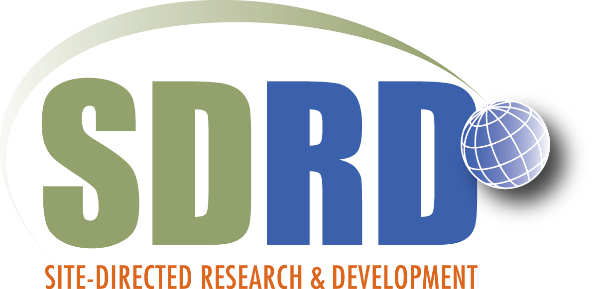
Project # 23-131 | Year 1 of 1
Michelle Scalisea, Eric Eckerta, Eric Matzelb, Arben Pitarkab
aNevada National Security Sites (NNSS), bNational Lab: LLNL
This work was done by Mission Support and Test Services, LLC, under Contract No. DE-NA0003624 with the U.S. Department of Energy, the NNSA Office of Defense Programs, and supported by the Site-Directed Research and Development Program. DOE/NV/03624–1884.
Abstract
Seismic hazard and nuclear explosion monitoring communities leverage physics-based modeling for ground motion prediction and source discrimination. These models enable analysis of wave generation and propagation by solving the elastic wave equation on three-dimensional grids, which can be computed to high frequencies with the use of High-Performance Computing systems. Accurate modeling at these high frequencies requires high resolution, realistic velocity models. The accuracy of such models depends on the quality of the geological and geophysical information, which often is not available at the required 3D resolution. Currently, waveform models utilize 3D geologic models to generate velocity models necessary for the calculations. The Nevada National Security Sites (NNSS)-developed Geologic Framework Models (GFMs) are among the most comprehensive 3D earth models in the country. While they accurately represent the large-scale 3D structure, they lack the small-scale spatial variation of seismic properties that are present in the shallow sedimentary layers. Our previous wave propagation modeling using the GFM has demonstrated that better characterization of the small-scale structural heterogeneity is needed to effectively propagate high frequency signals. We demonstrated the capability of using small scale seismic arrays to derive small scale variability that is not currently represented by the GFM.
Tie to Mission
The results from this project bring new technologies that use dense seismic arrays to generate high resolution tomography models, supporting the needs of many projects at the NNSS. Developing new methodologies for resolving material models at small spatial scales enhances capabilities in seismic monitoring and seismic hazard assessment. The Defense Nuclear Nonproliferation program is focused on understanding signals produced from evasive and low yield explosive sources. These high frequency sources are especially sensitive to scattering, which is largely controlled by high resolution heterogeneities in the underlying material. Results from this effort help characterize these materials, directly improving the far-field modeling capabilities that inform monitoring methodologies.
Technical Accomplishments
The primary goal of this feasibility study is to apply seismic tomography and interferometry techniques to existing small seismic array data. Seismic tomography is a method to image the subsurface velocity structure. Seismic signals from ambient noise, earthquakes, or active surveys are recorded by seismometers or geophones. Velocity is inverted by calculating the wave speed along each ray path between source and receiver. Traditionally, tomographic surveys are conducted over large regions with large station spacing to obtain smooth 3D models that image deep velocity discontinuities. The spatial resolution of the resulting tomographic inversion is a function of both the station spacing and frequency content of the recorded seismic energy. This study scales down the conventional geometries to resolve small spatial scale velocity heterogeneity over a limited geographic region at substantially higher resolution. The length scale and strength of velocity heterogeneities can inform the statistical properties of the correlated velocity perturbations applied to waveform simulations.
The resulting tomographic model was created using 24 hours of continuous data recorded by the 48 element mini array at Rock Valley deployed across an area of 100 x 100 m. Data for a single day (7/9/2023) was used to measure the response over a full day-night cycle, over which atmospheric and anthropogenic signals regularly vary, and to identify any complications that needed to be dealt with in processing. One of the stations had unaccounted for spikes in the data during this period, which caused erroneous results, but the remaining 47 had excellent signal well throughout the day and recorded a variety of environmental and ambient background noise.
We used interferometry to calculate empirical Green’s functions (EGFs) between each of the pairs of stations and measure the signal-to-noise response over frequency. From the 47 elements used we obtained 1081 EGFs at distances between 10 and 141 meters. The EGFs had excellent signal-to-noise between 5 and 50 Hz, allowing use of the full set for tomographic inversion.
The initial step was to invert for the best fitting 1D model along each of the EGF paths. This was done using a reflectivity code, simultaneously solving for P- and S-wave velocities (Vp, Vs) and frequency-dependent P- and S-wave quality factors (Qp and Qs). The objective function was to fit the synthetic seismograms to the envelope of the EGF over a set of 5 frequency bands divided evenly in log space. These 1D models were then combined to create the final 3D model.
Results demonstrate that small dense seismic arrays are capable of imaging 3D structure on length scales of 10s of meters. The results of this feasibility provide promising methodology to improve the resolution of 3D GFMs to meet waveform modeling needs.
Conclusions and Path Forward
This feasibility study has demonstrated that small seismic arrays can image small-scale velocity heterogeneity that is necessary to inform multiscale models. However, future work is needed to fully operationalize this capability. The stability and reproducibility of results needs to be explored through repeated seismic array deployments to verify that changes in ambient noise do not substantially change inversions. Deployments over varying lithostratigraphic units will generate heterogeneity distributions specific to different rock types. Effort needs to be taken to optimize seismic array geometry. The most challenging aspect of fully developing this capability is integrating the high-resolution model results from small seismic arrays into larger scale smooth GFMs. High resolution results can be analyzed for statistical distributions to apply to GFM model layers. Machine learning approaches may also provide promising ways of integrating multiscale models.
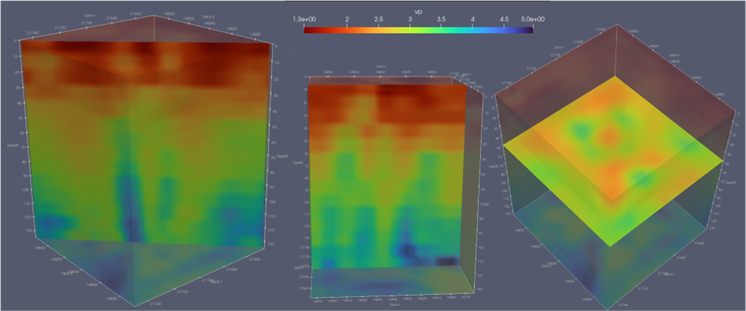