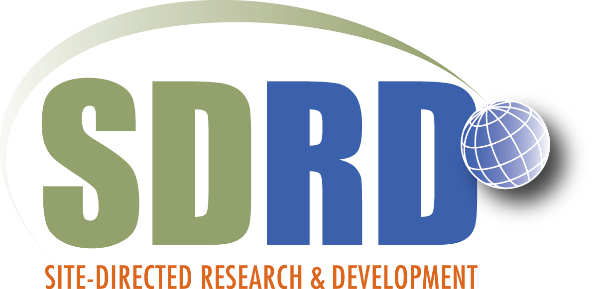
Imaging of Bubble Collapse Effects in Optically Transparent High Explosive as a Method to Study the Detonation Process
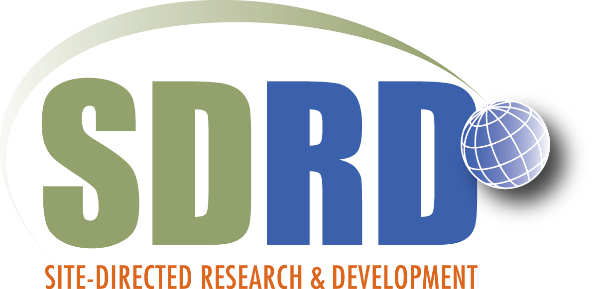
Project #: 22-051 | Year 3 of 3
Dale Turley, Brandon M. La Lone, Jason Mance, Matthew D. Staska, Gerald D. Stevens
Special Technologies Laboratory (STL)
Executive Summary
We used dynamic imaging and other techniques (velocimetry, radiometry, and spectroscopy) to investigate the processes leading to detonation in a homogeneous liquid explosive. By adding a gas bubble and observing its collapse caused by a shock wave propagating in the explosive, we were able to study the effect of “hot spot” formation during the early stages of the initiation process, furthering our understanding of explosive detonation.
Description
A polymer-bonded explosive (PBX) is a mixture of explosive granules and binder material characterized by zones of porosity and impedance mismatch. The pores are tens to hundreds of microns in size. Given this heterogeneity, when shocked, localized temperature impulses (hot spots) may occur due to void collapse and complex wave interactions. Hot spots can affect the reactive flow and rate at which some chemical reactions occur during the onset of detonation; however, the detailed contribution of hot spots to initiation of detonation is still not well understood. Our goal is to develop methods to investigate effects of hot spots on detonation mechanisms in explosives and apply experimental results to detonation simulation models. Because PBX is optically opaque, we selected nitromethane (NM), a transparent liquid explosive, for our studies.
In our prior work we used a small explosive charge to initiate detonation, added sensitizer diethylenetriamine to the NM to assist detonation, and added helium gas to form bubbles. All of these choices were identified as experimental limitations. In FY22, we improved our approach by replacing helium with butane, eliminating the sensitizer, and using a supported shock from a powder gun impactor to better control the shock state of the NM. We also modified our gas bubble experiment package to operate in the vacuum chamber of the STL powder gun. This experimental package allowed us to simultaneously image the shocked NM with a high-speed framing camera and measure material velocities, radiance, and spectroscopic signals. In a series of dynamic experiments, we were able to measure four important conditions of shocked NM: NM with and without a bubble, and with and without detonation. With our suite of measurement tools and this experimental approach, we were able to demonstrate the effect of a collapsing bubble on the explosive detonation process.
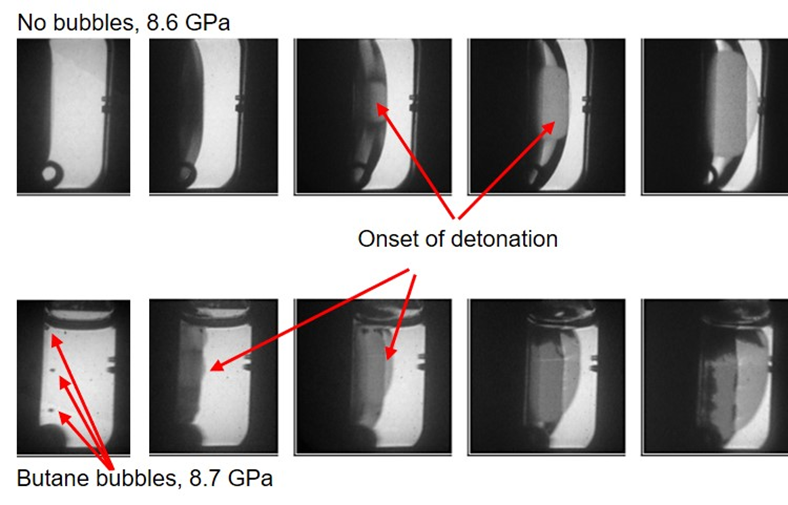
Conclusion
We investigated the incipient processes of detonation by studying shock-induced bubble collapse in NM. In this study, we quantified the effects of bubble collapse and peak input stress on the detonation process. We measured the time-resolved formation of hot spots and associated radiance temperatures and their effects on detonation using dynamic imaging, radiance, spectroscopy, and velocimetry measurements. Visible imaging identified distinct spatial features of detonation and, most importantly, the formation of a detonation wave. The simultaneous measurement of the emitted radiance and the time-resolved shock and detonation velocities shows temporal features well correlated with the detonation signature in the images. The signatures of detonation occurred significantly earlier in time when gas bubbles were present.
Mission Benefit
Using a butane bubble, we multi-diagnosed the phenomena of void-collapse and its influence on the detonation process. This process underlies many complex detonation models currently employed by the DOE, and, prior to our work, scant experimental evidence of hot spot behavior was published.
Hydrodynamic codes can model the localized energy increase caused by collapse of a void in an explosive and can provide insight into the effects of individual hot spots on detonation. Dynamic imaging of shock and detonation waves and hot spots can contribute to the understanding of wave dynamics and the fundamentals of the detonation process. Such measurements could be extremely useful in the understanding of reactive flow in the high explosive detonation burn process. Further understanding and control of hot spot densities can help tailor designs for high explosive detonation sensitivity and improve the predictive capability for sensitivity and initiation characteristics.
Publications, Technology Abstracts, Presentations/Posters
Turley W. D., B. M. La Lone, J. G. Mance, M. D. Staska, G. D. Stevens, L. R. Veeser, and D. M. Dattelbaum. 2021. “Experimental Observations of Shock-Wave-Induced Bubble Collapse and Hot-Spot Formation in Nitromethane Liquid Explosive.” J Appl Phys.129: 145102. https://doi.org/10.1063/5.0039414.
Turley, W. D., B. M. La Lone, J. G. Mance, M. D. Staska, G. D. Stevens, L. R. Veeser, and D. M. Dattelbaum. 2022. “Experimental Observations of Shock-Wave-Induced Bubble Collapse and Hot-Spot Formation in Nitromethane Liquid Explosive.” Manuscript in preparation.
This work was done by Mission Support and Test Services, LLC, under Contract No. DE-NA0003624 with the U.S. Department of Energy. DOE/NV/03624–1612.
Return to Dynamic Experiment Diagnostics
Go to SDRD Annual Report Index
Return to SDRD Homepage