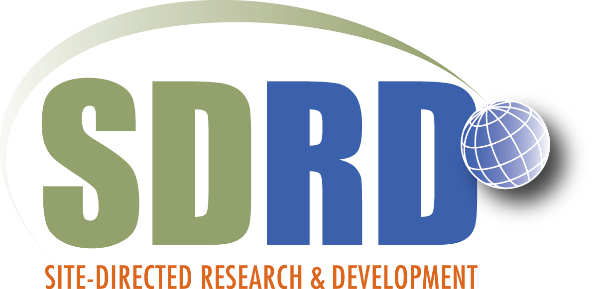
Enhancing Deep Cavity Detection Using Orthogonal Measurement Techniques
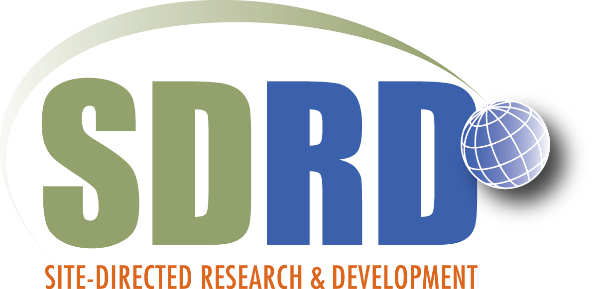
Project #: 22-074 | Year 3 of 3
Michael Howard, Melissa Wright, Heather Howard
Remote Sensing Laboratory-Nellis
Executive Summary
The ability to detect and characterize an underground facility (UGF) is a critical gap in our national capability. Modern mining techniques can reduce key signatures of tunneling, while only shallow tunneling has been effectively detected and characterized. Analysis of the spatial distribution of thermal inertia is a method that has been shown to detect shallow voids in the subsurface; however, to go deeper, we need to merge the capabilities of multiple measurement techniques. Supplementing surface temperature simulations with geophysical parameters obtained independently enhances a heat flow model so that a prediction can be made as to the times when the surficial thermal signature of a tunnel has the highest contrast with the host material. This enables improved targeting of thermal imaging assets to detect and characterize underground voids and facilities. The research integrates geophysical surveying with thermal inertia modeling; exploits orthogonal measurement techniques and airborne thermal imaging to detect voids; and has the potential to characterize the footprint of an underground facility and determine the limits on detection depths and sizes of subsurface voids.
Description
In order to detect thermal anomalies caused by subsurface voids, the surface energy balance and heat flow must be modeled to identify the time of maximum temperature separation between the void and host at the surface. At this maximum difference, a thermal anomaly from a subsurface void can be detected by overhead, time-sequenced, dual-band thermal imagery. Image processing techniques to reduce thermal clutter can be used to enhance the subtle thermal impact of subsurface structures on the surface emissions detected by remote sensing. In the first two years of this research, a thermal inertia model was developed that illustrated a measurable difference in the thermal contrast of the surface above a tunnel. In FY 2021, the model was expanded to include physical properties of the site geology, as well as surface weather data and downwelling solar radiation inputs, to characterize the surface energy balance more accurately. A sensitivity analysis was conducted to determine which input terms are the most significant to the model and how errors in the meteorological conditions, geologic material, and thermal properties affect the outcome of the modeled thermal contrast and predicted timing of maximum contrast. This study selected the Burrow Schmidt Tunnel (BST) located in Mojave, CA as a venue to investigate this detection method. In FY 2021, multiple programmatic issues limited the data collection opportunities at BST. This allowed for one Lawrence Livermore National Laboratory (LLNL) longwave-only hyperspectral imager (HSI) to be flown in July and one Canadian-based midwave infrared (MWIR)/longwave infrared (LWIR) HIS to be flown in September. During both airborne campaigns, ground truth operations collected surface temperature measurement on and off the tunnel, surface weather, tunnel air temperature, seismic and geologic surveys, measurement of thermal properties of the host material, and a GPS survey to map the tunnel trace for calculation of overburden along the tunnel. The seismic survey computed horizontal to vertical spectral ratio (HVSR) curves which were inverted to obtain a 3D subsurface model of velocity, layer thickness, and density. The vertical density profile further refined the model of subsurface heat flow distribution. In FY 2022, the project had additional programmatic problems with DOE aircraft availability that delayed the winter thermal HSI collection to late March and the data was not processed for analysis until July. Image processing proceeded to determine if the observed data collected by the HSI over the two seasonal periods could distinguish the subsurface tunnel trajectory. After using several analytical techniques, it was recognized that the image quality of the seasonal collections was not sufficient for this analysis approach; however, a review of the model parameters and prior empirical observations explain the findings.
Conclusion
Three significant technical achievements were made through this project despite the challenging programmatic issues. First, developing, refining, and implementing a thermal inertia model is a viable tool that demonstrated a method of predicting the optimum times for maximum thermal contrast to time MWIR/LWIR HSI data collections to detect a subsurface void. This was evaluated with surface radiometric and in situ weather ground truth. Performance of sensitivity analyses during simulations discovered thermal and material properties of the host geology are critical terms that can drastically affect the temporal and thermal contrast predicted by the model. However, properties related to weather and surface characteristics, like air temperature, surface emissivity, and albedo, do not affect the imaging timing but are factors in getting accurate estimates for thermal contrast. This substantiated the second achievement in the development of a geologic framework for the study site. This was derived from mapping of the surface geology and a seismic survey at BST which defined the thermal diffusivity term by providing an accurate vertical distribution of rock density. The third achievement was comparing the model predictions regarding the optimum collection times and analyzing differences from the actual observed collection times. These results indicated that the airborne data collections were not optimally timed to produce the maximum and minimum thermal contrast in surface temperature. This disadvantage was great enough that the observed data could not support the intended analysis. In addition, analysis revealed that the noise equivalent differential temperature (NEDT) of the Itres sensors were higher than expected which did not allow for the measurement of the subtle target signatures.
Mission Benefit
This research had direct relevance to the NNSA office of Defense Nuclear Nonproliferation (DNN) mission as well as to the greater United States proliferation detection community. The outcome of this project benefits the NNSA and other national and domestic security stakeholders by providing thermal modeling tools that predict heat flow through a geologic column and the use of geophysical survey techniques to provide an orthogonal data to enhance that model. This research will further the utility and applications of remote sensing as a means to detect and characterize UGFs as it would be directly transferrable to airborne and satellite-based systems for monitoring domestic borders and denied territories.
This work was done by Mission Support and Test Services, LLC, under Contract No. DE-NA0003624 with the U.S. Department of Energy. DOE/NV/03624–1655.
Return to Enabling Technologies for Autonomous Systems and Sensing
Go to SDRD Annual Report Index
Return to SDRD Homepage