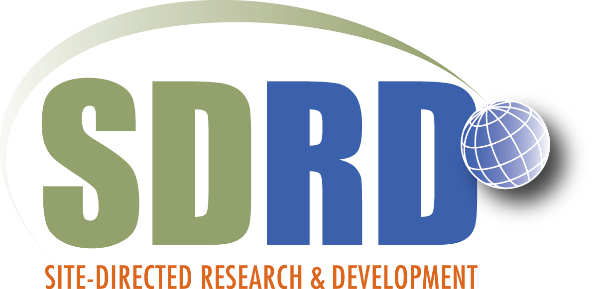
Detector Wall Research for Fast Gamma Signal Detection in Neutron-Diagnosed Subcritical Experiment Applications
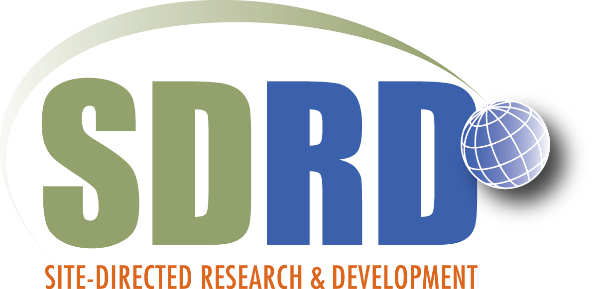
Project #: 22-045 | Year 3 of 3
Stuart Baker,a David Schwellenbach,a Charles Leak,a Robert Buckles,a Amber Guckes,a Richard S. Woolf,b Bernard Philipsb
aNevada National Security Site (NNSS); bNaval Research Lab (NRL)
Executive Summary
This project pursued a new gamma radiation detection method with evaluation of a detector concept designed for ultra-high sensitivity along with fast (ns) time response. The intended application is high accuracy measurement of a pulsed gamma signal produced in Neutron-Diagnosed Subcritical Experiment (NDSE) tests. Gamma time profile measurement accuracy is crucial for determining the nuclear reactivity and criticality of NDSE tests.
Description
The NDSE testing platform capability is the next generation of instrumentation for subcritical nuclear weapon testing being planned for the Zeus testbed in the U1a facility. A gamma-ray detection system with fast (5 ns) time response is required to qualify initial neutron induced reactivity measurements of the NDSE test. Initial NDSE tests will be executed with a baseline programmatic detector system. Our research pursued a new detector solution for future NDSE tests that indicate a need for better sensitivity and time response. A more efficient detector system would provide better measurement statistics in a more compact configuration and provide flexibility to experimental configurations.
The baseline detector design utilizes 151 detector elements to make up a large ~3-meter diameter gamma detector wall. A large area detector wall is needed to achieve good gamma measurement statistics from a 20-meter stand-off distance. The stand-off distance provides time-of-flight separation of incoming gamma and slower neutron signals. The detector was designed programmatically with the NNSS and Los Alamos National Laboratory (LANL) teams based on the current state-of-the-art components at the time to qualify this criticality measurement method for NDSE program.
Our need for high gamma sensitivity led us to test a new method for scintillator light collection. In our first trial we utilized wavelength shifting (WLS) optical fibers inserted into an array of long scintillator cells to couple the gamma signal scintillator light to a small fast photomultiplier tube (PMT). Narrow detector cells minimize time spread of the scintillation light in the detector volume. Scintillator light is collected through the length of the detector with WLS fiber for efficient signal coupling to the PMT. Single optical fibers from multiple detector cells are bundled to a single PMT for high detection sensitivity. Detector tests were performed at the NNSS Los Alamos Operations (LAO) linear accelerator (LINAC) for MeV x-ray impulse signal time response measurements. Prototype detectors were tested in the same geometry and x-ray flux as early prototype NDSE detectors. This test series provided detector time response and relative sensitivity comparison to the baseline NDSE detector design.
Tests were first performed on a small-format 5 cm x 5 cm prototype detector. This detector proved to be highly sensitive while maintaining fast time response. Detector response was measured in PMT signal to be approximately 20 times more voltage per scintillator area and volume over the large area NDSE baseline prototype. However, to be comparable to the large-area baseline detector wall element, the scintillator area and volume must be scaled up to provide relative statistical detection efficiency measurements. Differences in response between the baseline PMT and WLS fiber-optically coupled PMT must also be considered and accounted for.
To produce a detector on the scale of the baseline pixel, our team worked with NRL to scale up the dimensions of the design to a 20 cm x 20 cm area detector. We subcontracted larger extruded scintillator fabrication through Fermi National Laboratory. Three detector configurations were then tested by the NNSS Detectors group for optical PMT response and sensitivity in the NNSS Source Range with a Cobalt-60 gamma source. Test results show that the smaller format detector configuration proved to be approximately 10 times more sensitive than the larger format detector.
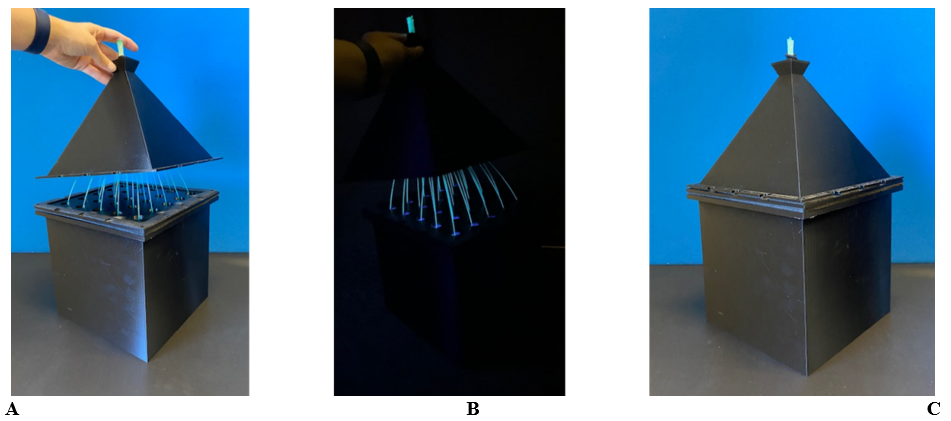
Conclusion
The small format detector is shown to be highly sensitive for MeV gamma radiation detection with good time response. The small format detector scheme proved to be highly efficient and could prove to be highly useful for certain x-ray and gamma signal monitoring, as well as neutron time-of-flight. To be field ready the small format detector would benefit with an optimization effort from the NNSS Detectors Group.
The large format detector evaluated was limited in sensitivity with poor optical collection efficiency that would need to be remedied for further pursuit of a scaled up large area on the order of the NDSE detector wall. There are several areas to consider for optimization, such as more optical coupling WLS fiber to a larger PMT. Further investigation of the large format type detector wall concept would take a focused effort by the NNSS Detectors Group to remedy the trouble spots. There is some further detailed discussion in the reference Buckles et al. (2022).
Mission Benefit
This research investigated new methods of optical signal coupling for none-imaging radiation detectors, and methods to improve gamma signal detection sensitivity and efficiency. NDSE tests planned for U1a will use fast gamma detectors to measure of dynamic reactivity to infer criticality of the test. Highly accurate gamma response measurements are essential to minimize uncertainty to inform predictive models and dynamic performance of device design.
Publications, Technology Abstracts, Presentations/Posters
Woolf, R. S., B. F. Philips, S. A. Baker, A. L. Hutcheson, R. Perea, D. D. Schwellenbach, E. A. Wulf. 2021. “Development of an Extruded Plastic Array for Narrow Scintillation Pulse Widths.” Nucl Instrum Methods Phys Res A.1002: 165283. https://doi.org/10.1016/j.nima.2021.165283
References
Buckles, R., J. Mellott, A. Wolverton, I. Garza, S. Baker. 2022. “Evaluation of NRL Arrayed-Scintillator Detectors as NDSE Candidates.” Internal project report, TBP. Las Vegas, Nevada: Mission Support and Test Services, LLC.
Woolf, R., C. Leak. 2022. “Deliverable for Year 3 (Task 1, 2, and 3).” Internal project report. NRL, TSC-Praxis.
This work was done by Mission Support and Test Services, LLC, under Contract No. DE-NA0003624 with the U.S. Department of Energy. DOE/NV/03624–1624.
Return to Neutron Technologies and Measurements
Go to SDRD Annual Report Index
Return to SDRD Homepage