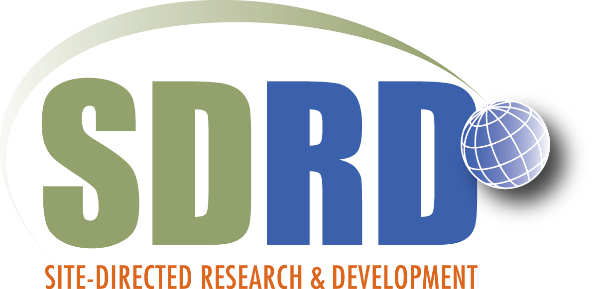
Dynamic Sub‐Micron Particulate Behavior in Turbulent Media
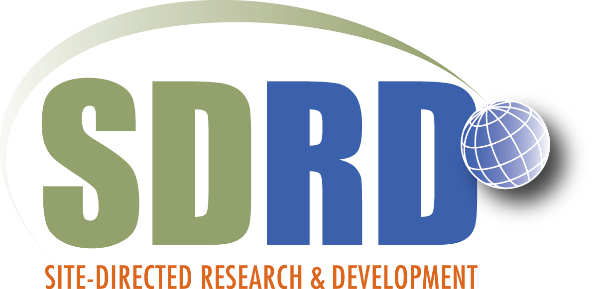
Project #: 22-046 | Year 3 of 3
Clare Kimblin,a Roy Abbott,a Manuel Manard,a Ian McKenna,a Thomas Myers,a Matthew Staska,a Paul Taylor,a Mary O’Neill,b Jason Sears,c Jens von der Linden,c Josef Dufek,d Joshua Mendez Harperd
aSpecial Technologies Laboratory; bKeystone International; cLawrence Livermore National Laboratory; dUniversity of Oregon
Executive Summary
To investigate RF and charge associated with turbulently mixed carbon particles and so inform modeling of large-scale detonation signatures, we have performed three types of novel experiments using new experimental platforms. In doing so we have captured conditions which favor streamer formation with shocked diamond powder, demonstrated that triboelectrically charged carbon detonation particles (CDPs) produce RF emissions, and have confirmed that CDPs produced within .5 ms following a detonation are charged.
Description
Particulates form nearly instantly following a detonation and can provide clues as to the high explosive (HE) device that produced them. Carbon soot and metal/oxide particulates are thought to influence real time RF emissions, and particulates collected post-event serve as long lasting forensic evidence. To inform modeling of detonation signatures associated with particulates, we have performed low technology readiness level (TRL), controlled experiments using platforms designed to interrogate aspects of HE detonation signature production. Using these, we determined conditions and C allotropes that favor production of RF emissions, tested the theory that CDPs produce significant RF emissions, and explored charge on DPs produced within a ms following a detonation.
When released from the Shock Tube Overpressure Apparatus (STOA), diamond produces significant observable discharges that are initially confined by the pressure gradient at the perimeter of a standing shock wave (von der Linden et al. 2021). Until this year, we had not confidently demonstrated that CDPs produce electrical discharges under conditions which isolate triboelectric charging. The observation that diamond produces significant discharges while detonation soot and graphite produce only a few (detonation soot), or no observable discharges (graphite), is in line with fluidized bed and vibrating ramp studies at the University of Oregon. In fluidized bed and vibrating ramp studies, it was observed that electrically insulating diamond (125-250 µm nominal grain size) sustains a broader bipolar charge distribution than Composition B (CompB) detonation soot (DS) and conductive graphite (125-250 µm nominal grain size). Under this project, using optimized diagnostic and analysis techniques, we have recorded coincident RF and optical pulses (Figure 1A) indicating that discharges are produced when CDPs (both those comprised of graphitic soot and those comprised of nanodiamond with graphitic soot) are released from an Al shock tube (Figure 1B). Emissions up to 4 GHz were recorded (Figure 1C).
To determine whether RF emissions were more prominent with a soot producing HE formulation vs. one which was oxygen balanced, we compared RF emissions from a fuel rich HE formulation, known to produce a significant amount of graphitic soot, to RF from an oxygen balanced nitromethane formulation. For this we used the 1 m3 polycarbonate (PC) RF chamber shown in Figure 1D. Preliminary results indicate that in the first millisecond following detonation, the fuel rich formulation yielded more RF pulses (Figure 1E). Repeatability has not yet been established, however. As with the shock tube experiments with soot, RF emissions up to 4 GHz were also recorded.
Particulate charge, and mass to charge ratio (m/z) are relevant to understanding RF generation and particulate aggregation, but the charge on individual detonation particles has not been measured immediately following a detonation. This year we performed experiments to determine the feasibility of measuring m/z on particles produced within a millisecond following a detonation. Using the system shown in Figure 1Gour results (Figure 1F) indicate that charged particles are detected. This is the first step leading toward using a time-of-flight (TOF) mass spectrometer (MS) (built under STL-17-11 “Miniaturizing time-of-flight mass spectrometers”) to directly characterize detonation particles. Under 23-007, we will use the time of arrival of particles at the Channeltron electron multiplier (CEM) detector, the electric field of the reflectron, and distance between the reflectron and the detector, to calculate m/z. Using m/z and mass inferred from particle size, we will estimate particle charge. Alternatively, using direct measurement of particle charge, we will infer particle mass.
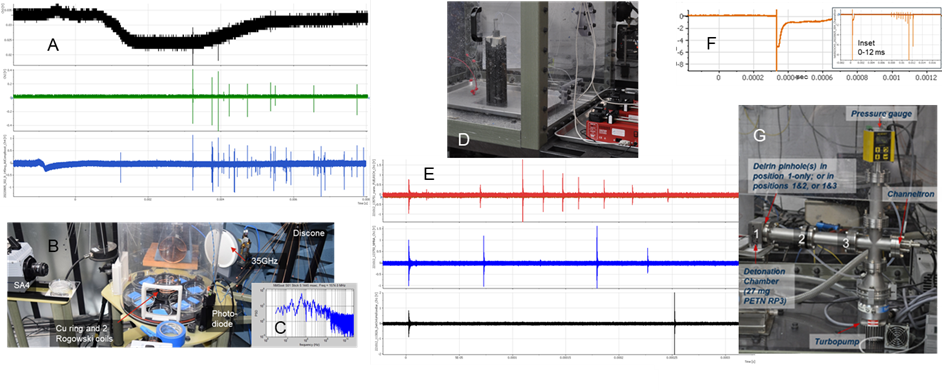
Conclusion
Using platforms, diagnostics, and an analysis code developed under this SDRD, we have investigated the effect that triboelectric charging has on prompt emissions from known particulates. We observe significantly more RF and optical emissions with electrically insulating diamond relative to conductive graphite and detonation soots, but have demonstrated that CompB and TNT detonation soots produce RF and concurrent light emissions when ejected from the Al shock tube. In preliminary nitromethane experiments, more RF emissions were observed in the first 1 ms following detonation of a fuel rich HE formulation vs. detonator alone, and vs. oxygen balanced formulation, but further repetition is required to ascertain variability. Overall, our results demonstrate that the triboelectric charging of detonation soots does result in RF emissions, but we have not compared emissions from the triboelectric charging of soot to that from other detonation materials, or to mixtures of materials. Using a CEM and small PETN [pentaerythritol tetranitrate] detonation we have demonstrated that charged particles are produced, and the feasibility of making a TOF mass spectrometry measurement under STL-007-23.
Mission Benefit
This work provides new NNSS capabilities in the form of experimental platforms, diagnostics, and analysis code. The particulate shock tube permits verification of optical as well as hydrodynamic and electrostatic models (as described in Von der Linden et al.). Using the shock tube, we have demonstrated that triboelectric charging of carbonaceous soot does generate RF emissions. The role of metal in shock tube and RF chamber experiments requires more investigation. In FY 2023, the shock tube and the PC RF chamber will be used to perform small scale experiments in support of three non-proliferation projects. In addition to providing platforms for small-scale experiments, the two platforms also permit testing of diagnostics and analysis techniques prior to fielding equipment at large scale tests. The Data Analysis code, first developed to analyze RF and high-speed camera imagery from the shock tube, is now being leveraged by multiple projects for field data analysis. Finally, we have also demonstrated that measurement of m/z for detonation particles produced directly from a detonation will be feasible. We will continue the m/z investigation under STL-007-23.
Publications, Technology Abstracts, Presentations/Posters
Manuscript demonstrating consistency between experiment and hydrodynamic & electrostatic models: von der Linden, et al. 2021. “Standing shock prevents propagation of sparks in supersonic explosive flows.” Communications Earth & Environment. 2: 195. doi: 10.1038/s43247-021-00263-y.
O’Neill, M., Tech Note MDO2019-TN010 R3, “RF Analysis Code User Guide,” 14 May 2020 (document is OUO); revisions have been made in subsequent tech notes and guides, this is revision 3 following the inception of the data analysis code.
O’Neill, M., Data Analysis User Manual R5a, “Data Analysis Tools User Guide”, 1 December 2022 (document is OUO).
Mendez-Harper, J., C. Kimblin, I. McKenna, J. Dufek. Manuscript. “Tribolelectric Charging of Carbon Allotropes.”
von der Linden, J. 2020. “Standing Shock Regulates Sparks in Explosive Flows.” Presentation [virtual] given at the 73rd Annual Meeting of the APS Division of Fluid Dynamics; Chicago, IL.
von der Linden, J. “The Effect of Particles on Standing Shockwaves Regulating Spark Discharges in Volcanic Eruptions.” Presentation given at 61st Annual Meeting of the APS Division of Plasma Physics; Fort Lauderdale, FL.
von der Linden, J. 2019. “The Effect of Particles on Standing Shockwaves Regulating Spark Discharges in Volcanic Eruptions.” Poster presented at the 2019 Fall AGU Meeting; San Francisco, CA.
References
Manard, M. J. 2011. “Miniaturizing Time-of-Flight Mass Spectrometers.” In FY2011 Site-Directed Research and Development Annual Report, 139-150. Las Vegas, Nevada: National Security Technologies, LLC.
von der Linden, J. et al. 2021. “Standing shock prevents propagation of sparks in supersonic explosive flows.” Communications Earth & Environment. 2: 195. doi: 10.1038/s43247-021-00263-y.
This work was done by Mission Support and Test Services, LLC, under Contract No. DE-NA0003624 with the U.S. Department of Energy. DOE/NV/03624–1613.
Return to User-Centered Remote Testing and Operations
Go to SDRD Annual Report Index
Return to SDRD Homepage