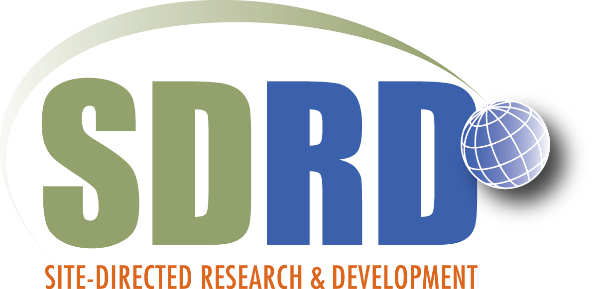
Fundamental Experiments for Detonation Signature Modeling
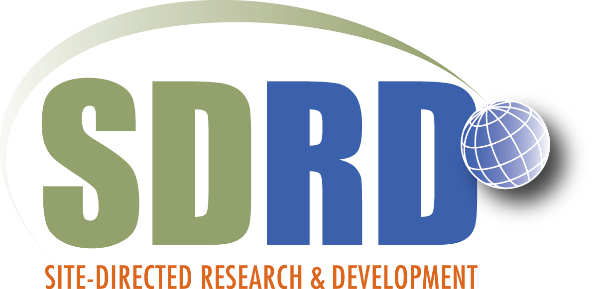
Project # 23-007 | Year 1 of 3
Clare Kimblina, Roy Abbotta, Sean Brecklingb, Manuel Manarda, Thomas Myersa, Ian McKennaa, Tristan Richmonda, Cliff Watkinsa, Mary O’Neillc, Professor Josef Dufekd, Joshua Mendez Harpere, Michelle Zimmermanf
aSpecial Technologies Lab (STL), bNevada National Security Sites (NNSS), cKeystone, dUniversity of Oregan, ePortland State University, fUniversity of California, Santa
Barbara
This work was done by Mission Support and Test Services, LLC, under Contract No. DE-NA0003624 with the U.S. Department of Energy, the NNSA Office of Defense Programs, and supported by the Site-Directed Research and Development Program. DOE/NV/03624–1946.
Abstract
Our goal is to perform low technical readiness level (TRL) experiments that ultimately lead to improvements in understanding signatures produced by particulates in overpressurized, supersonic flows. We are taking a multipronged approach: exploring prompt radio frequency (RF) emissions and optical extinction as a function of particle type, comparing RF emissions from oxygen balanced vs. fuel rich detonations, and attempting to address gaps in particle evolution models by measuring carbonaceous particle mass-to-charge ratios (m/z) milliseconds post-detonation. This year we have measured RF and optical emissions produced by a range of particles with known conductivity and permittivity as they are released from the Special Technologies Laboratory (STL) shock tube. The RF measurements indicate that, in general, with increased conductivity the production of discharges is reduced. However, we find that high atomic number hard metals also produce discharges, and that an electrically insulating but soft material produces very few. Flake vs. powder studies indicate that metal particle morphology is also important. Simulations of the base of the shock tube improve our understanding of the vorticity and turbulent kinetic energy that results in inter-particle mixing. With the addition of glass micro-balloons, we have achieved detonation with fuel-rich vs. oxygen balanced nitromethane formulations. RF emission density appears to be slightly higher with the fuel rich formulation. This year we have successfully paired an HE detonation chamber (DC) with a time-of-flight mass spectrometer (ToF MS). Using this system, very preliminary results indicate that particles with mass to charge ratio in the 3000-4000 amu range are detected.
Background
Detonation particles (DPs) are responsible for prompt RF emissions and optical effects, and residual particles can be used forensically. Understanding how conditions and signatures are related requires further study, however.Using small-scale, low TRL, controlled experiments involving a particulate shock tube, mass spectrometry, and small-scale detonations with a liquid explosive, we seek to provide fundamental tools and data to inform RF, optical, and particulate evolution models.
With the shock tube we aim to better understand the fundamental behavior of particles as a function of known quantities that regulate both their ability to become charged and discharge, and their absorption and scattering of light. Maxwell’s equations dictate some of this behavior. In Maxwell’s equations a key parameter is the dielectric constant, equal to the square of a material’s complex index of refraction (n = n+ik). The real part (pseudo permittivity for non-magnetic materials) is related to the degree to which a material can become polarized by an electric field, and the imaginary part (pseudo conductivity) is the extinction coefficient. Particle hardness, tensile strength, and surface area are also of interest because they may influence particle charging and RF emissions via triboelectric, fracto-charging, and Misra effects. To our knowledge this is the first series of multi-material studies of this type.
The charge on core carbon nanoparticles produced by a detonation may influence the charging of particles they are in contact with and may influence the propensity of the core particles to aggregate. We are pairing an HE detonation chamber with a mass spectrometer as a means to explore mass and charge of detonation particles produced milliseconds post-detonation. To our knowledge this has not been done before.
Technical Approach
Using the STL shock tube (developed under STL-024-17 and STL-008-20) we are exploring the impact that fundamental particle characteristics have on discharge production and optical obscuration. Particles chosen range broadly in hardness, fracture toughness, permittivity, and conductivity. Flake vs. powder morphology was also explored. This year the focus has been on RF emissions. RF (kHz to GHz) and optical (high speed camera and photodiode) measurements were used to capture the propensity of the ejected particles to produce discharges. A Faraday cup array was built and evaluated to determine how well charge on particles can be measured as they are ejected from the shock tube, and new supersonic gas modeling capabilities were stood up. 2D vorticity and momentum magnitude simulations led to improved comprehension of the turbulent mixing and scouring of particles at the base of the shock tube, where gas enters perpendicularly. We also began to explore the use of a high-speed slit-spectrometer, to potentially improve our mechanistic understanding of discharge production.
Using the STL shock tube (developed under STL-024-17 and STL-008-20) we are exploring the impact that fundamental particle characteristics have on discharge production and optical obscuration. Particles chosen range broadly in hardness, fracture toughness, permittivity, and conductivity. Flake vs. powder morphology was also explored. This year the focus has been on RF emissions. RF (kHz to GHz) and optical (high speed camera and photodiode) measurements were used to capture the propensity of the ejected particles to produce discharges. A Faraday cup array was built and evaluated to determine how well charge on particles can be measured as they are ejected from the shock tube, and new supersonic gas modeling capabilities were stood up. 2D vorticity and momentum magnitude simulations led to improved comprehension of the turbulent mixing and scouring of particles at the base of the shock tube, where gas enters perpendicularly. We also began to explore the use of a high-speed slit-spectrometer, to potentially improve our mechanistic understanding of discharge production.
To address gaps in charging and particulate evolution, we paired a DC to a ToF MS (built under STL-17-11 ). A typical mass spectrometer where ions are generated by laser ablation or other traditional means requires an ion funnel to maximize transmission of ions through the system, and an accelerating electric field. We instead use a ~27 mg PETN detonator as the source of clustered carbon ions. These high kinetic energy species do not require additional guidance and acceleration to travel from the source to the detector. In the ToF MS paired with the DC, a reflectron/ion mirror provides a repulsive electric field that reduces the spread in KE for identical m/z ions. This permits detection of particles with higher time resolution. From the particle times of arrival at the Channeltron detector, the known pathlength traveled, and the reflectron electric field it is possible to calculate m/z of the particles generated. SIMION® was used for this purpose.
It has been proposed that RF emissions are significantly influenced by carbon soot, but this has not been demonstrated using formulations that are nearly identical. To test this theory, we compare RF produced by a fuel rich nitromethane formulation (95:5 v/v NM:NA) to RF from an oxygen balanced nitromethane formulation (68:32 v/v NM:NA). To achieve detonation, it was found that glass micro-balloons had to be added to the liquid formulations. They accelerate the shock front via hot-spot creation.
Results and Technical Accomplishments
Shock tube experiments were performed using 15 powders with a broad range of properties. In general, we observed that fewer discharges are detected as the electrical conductivity of the particles increases. However, high atomic number, less deformable metals appeared to produce more discharges than more deformable metals; also, soft, but insulating, Carnauba wax produces negligible RF. We see that particle morphology impacts RF production also. With moderately hard and ductile stainless steel (SS 316 L) powder discharges are not detected, but with SS 316 L flake numerous discharges are detected. Our understanding of the forces that act on the particles within the shock tube has improved due to steady state simulations of gas velocity, pressure, and density fields. Due to the 90-degree bend at the gas inlet, supersonic KH instabilities are observed due to strong vertical shear between gas at different pressures; and regions of high shear are distinct from regions with high pressure gradients.
We were successful in pairing a DC and 27 mg PETN detonator with a ToF MS. Under initial conditions the reflectron and CEM power supplies consistently tripped following detonation. We determined that detonation produced sufficient pressure to produce breakdown at the high voltage power supplies. To remedy this, we increased the volume of the DC and actively pumped on it. Once remedied, we observed ringing signal, possibly attributable to mechanical vibrations associated with shock, in the 2- to 7-ms range, and less intense downward-going signal in the 100 s range. Modeling of the experiment with SIMION® indicates that singly charged 1.5 to 15 million amu particles yield flight times in the 2- to 7-ms range, whereas singly charged 3000-4000 amu particles yield flight times in the 100 s range. Core detonation particles (predicted to be ~10,000 C per ~5 nm nanoparticle) are expected to aggregate within 10 microseconds following detonation. In FY24, experiments will be performed to determine the origin of the ringing signal.
With the liquid HE soot/no-soot experiments we demonstrated that detonation occurs reliably with glass micro-balloons present and that the presence of soot appears to result in a denser grouping of RF pulses. Fractured micro-balloons however provide another source of particles and complicate the experiment.
Conclusions and Path Forward
Shock tube experiments were performed using 15 powders with a broad range of properties. In general, we observed that fewer discharges are detected as the electrical conductivity of the particles increases. However, high atomic number, less deformable metals appeared to produce more discharges than more deformable metals; also, soft, but insulating, Carnauba wax produces negligible RF. We see that particle morphology impacts RF production also. With moderately hard and ductile stainless steel (SS 316 L) powder discharges are not detected, but with SS 316 L flake numerous discharges are detected. Our understanding of the forces that act on the particles within the shock tube has improved due to steady state simulations of gas velocity, pressure, and density fields. Due to the 90-degree bend at the gas inlet, supersonic KH instabilities are observed due to strong vertical shear between gas at different pressures; and regions of high shear are distinct from regions with high pressure gradients.
We were successful in pairing a DC and 27 mg PETN detonator with a ToF MS. Under initial conditions the reflectron and CEM power supplies consistently tripped following detonation. We determined that detonation produced sufficient pressure to produce breakdown at the high voltage power supplies. To remedy this, we increased the volume of the DC and actively pumped on it. Once remedied, we observed ringing signal, possibly attributable to mechanical vibrations associated with shock, in the 2- to 7-ms range, and less intense downward-going signal in the 100 s range. Modeling of the experiment with SIMION® indicates that singly charged 1.5 to 15 million amu particles yield flight times in the 2- to 7-ms range, whereas singly charged 3000-4000 amu particles yield flight times in the 100 s range. Core detonation particles (predicted to be ~10,000 C per ~5 nm nanoparticle) are expected to aggregate within 10 microseconds following detonation. In FY24, experiments will be performed to determine the origin of the ringing signal.
With the liquid HE soot/no-soot experiments we demonstrated that detonation occurs reliably with glass micro-balloons present and that the presence of soot appears to result in a denser grouping of RF pulses. Fractured micro-balloons however provide another source of particles and complicate the experiment.
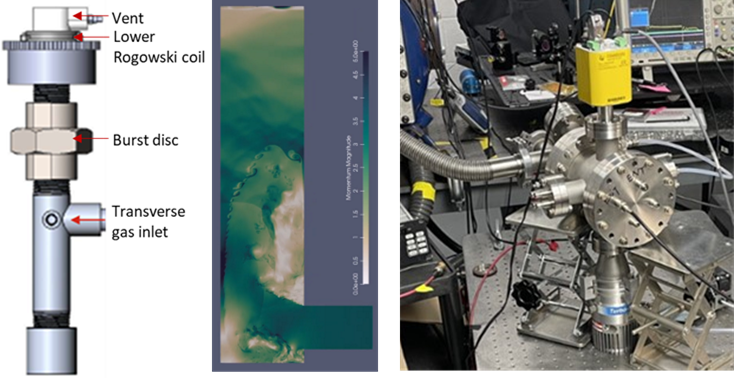
Publications
- Title: Electrostatic Discharges in Explosive (supersonic) Multiphase Flows
Journal / Conference: Electrostatics Society of America conference
Year: 2023
Author(s): Joshua Mendez Harper, Clare Kimblin (Co PIs), Josef Dufek, Ian McKenna, Corrado Cimarelli, Tristan Richmond