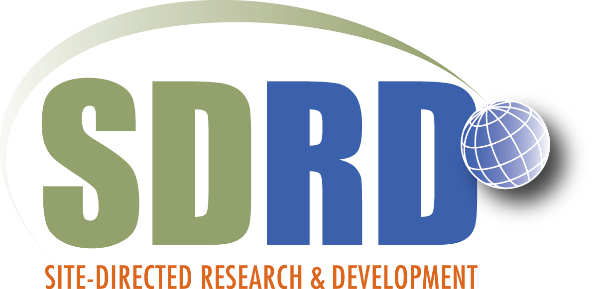
Homogenous Detonation of High Explosive by High Intensity Non-Coherent Radiation
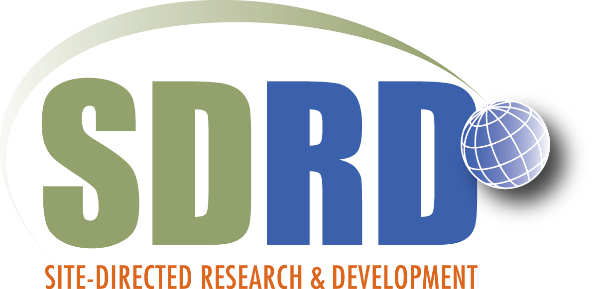
Project # 23-070 | Year 1 of 3
Thomas Myers, Chris Kayda, Eric Larson, Brandon La Lone, Jason Mance, Matt Staska, Gerald Stevens, Dale Turley, Ruben Valencia
Special Technologies Lab (STL)
This work was done by Mission Support and Test Services, LLC, under Contract No. DE-NA0003624 with the U.S. Department of Energy, the NNSA Office of Defense Programs, and supported by the Site-Directed Research and Development Program. DOE/NV/03624–1934.
Abstract
This project sought to develop a homogenous explosive drive by combing a shocked xenon light source with a liquid binary explosive mixture. The development of the shocked xenon light source was successful and sustained a blackbody temperature of at least 25,000 K for 15 microseconds but was unable to detonate mixtures of concentrated nitric acid with various organic fuels. While reported to have extreme sensitivity towards shock, impact, and heat, liquid explosive mixtures of nitric acid and fuels failed to detonate even when shocked with a conventional exploding bridgewire detonator. In light of this, other explosives were investigated, and while the conventional secondary explosive pentaerythritol tetranitrate (PETN) could not be optically detonated with a shocked xenon light source, the experimental primary explosive DBX-1 was successfully detonated. However, due to the limitations on contact work with primary explosives, continuation of this success at the Nevada National Security Sites (NNSS) is not possible at this time. Additionally, this project developed several new capabilities that have benefited multiple NA-10, NA-20 and Site-Directed Research and Development (SDRD) funded projects including an optically/radio frequency (RF) transparent explosive containment vessel and the safety basis to perform high hazard small scale explosive operations.
Background
Experiments requiring planar explosive drives rely on the use of explosive lenses. Typically, a low detonation velocity explosive is encased within a high detonation velocity explosive such that when initiated from a common point, the shock waves arrive simultaneously at the output plane. Alternatively, inert wave shapers can be embedded in explosive charges to generate similar planar shock outputs. While these methods are successful at generating an initially planar shock, the pressure waves behind the shock are non-uniform and the symmetry is broken. Additionally, these approaches often require more than 1 kg of total high explosive and precision machining of multiple explosive parts, making them too costly and complex for laboratory scale shock physics experiments.
More recently, alternative approaches have been taken to reduce the mass of explosive required while also improving the performance of the system. Specifically, 3D-printed explosives and wave shapers have been employed with some success to generate small planar shock waves utilizing far lower explosive masses. However, voids and irregularities in the cast explosive utilized led to a breakdown of the shockwave planarity.
Alternatively, explosives initiated with light may provide several advantages over conventional explosive drives. Light is capable of heating and initiating the entire surface of an explosive charge nearly simultaneously. This allows for true 1D shocks to be achieved where both the arrival of the shock front and the pressure profile behind it are uniform across the entire charge. Several different explosive materials have been shown to detonate under laser irradiation including PETN and several primary explosives. While lasers are effective means of initiation, they are difficult to scale to larger geometries due to the cost and complexity of generating higher energy laser pulses. In contrast, incoherent light sources such as flashlamps are more easily scaled. There are a few reports of incoherent light being used for detonation of the most sensitive primary explosives, but the extreme sensitivity and exotic nature of the primary explosives involved limits the utility of this approach.
Technical Approach
To avoid the danger of contact operations with primary explosives, this project investigated liquid binary explosives. Specifically, mixtures of concentrated nitric acid with fuels such as nitromethane and nitrobenzene have been reported to have extreme sensitivity to external stimuli (impact, spark, heat, etc.), small critical diameters, and greatly enhanced shock sensitivity. These mixtures can be remotely mixed to avoid the need for contact operations as well as rendered inert through dilution with water. Paired with a bright incoherent light source, these liquid binary explosive mixtures should provide an ideal explosive for optical initiation.
To generate high power bursts of incoherent light, this project selected shocked xenon light sources. When shock waves pass through noble gases such as argon or xenon, the gases are compressively heated to extremely high temperatures and emit intense blackbody radiation in the visible and ultraviolet regions of the spectrum. The intensity and duration of the light is dependent on the composition, mass, and geometry of the explosive charge providing the shock. The simplicity and unmatched light output from these light sources made them ideal candidates for optical initiation of liquid binary explosive mixtures.
Development of a shocked xenon light source focused on maximizing the optical fluence delivered to the explosive target plane. This element of the project focused on optimizing the distance between the explosive charge, the target plane, the mass of explosive used as the source of the shockwave, and the configuration of high reflective foils. To determine the temperature and emissivity of the shocked xenon light source, a 6-channel pyrometer was built and calibrated. Each small charge was detonated, and the light output characterized with the pyrometer until an optimized design was determined.
To address the second element of the project, the safe handling of mixtures of nitric acid with organic fuels, a new explosive containment vessel was constructed to allow for handling of concentrated nitric acid-based explosive mixtures. Due to the unique hazards of concentrated nitric acid, it was necessary that the containment vessel used for this work contains not only the explosive hazards (blast, fragments, fireball, etc.) but also the chemical hazards of the nitric acid (corrosive, oxidizing, reactive, etc.). After reviewing the compatibility of nitric acid with various materials, it was determined that a polycarbonate construction would provide both mechanical strength as well as chemical resistance.
In addition to the need for a new containment vessel to address the hazard of the concentrated nitric acid, equipment for the remote mixing of the binary liquid explosive mixtures was required. A system utilizing remotely controlled syringe pumps allowed for the remote mixing of the liquid components. Additionally, the system could flood the binary liquid explosive mixture with water, which diluted the nitric acid and rendered the mixture non-explosive. Experiments were conducted with the optimized shocked xenon light source and the liquid explosive mixtures using high speed imagery and photonic Doppler velocimetry to determine if detonation had occurred. Other explosives were also tested in the same setup to see if optical initiation of secondary explosives (PETN) and primary explosives (DBX-1) could be achieved.
Results and Technical Accomplishments
This project was successful in developing an optimized shocked xenon light source capable of maintaining a blackbody temperature of at least 25,000 K for 15 microseconds. Additionally, the project developed a chemically resistant RF/optically transparent explosive containment vessel to support safe operations with explosive mixtures of nitric acid and fuels. The team also developed a remote-controlled syringe system capable of cradle to grave remote operations with liquid primary explosives, allowing this project to become the only explosive hazard class – I and non-contact primary explosive operation currently conducted at the NNSS.
However, the project was unable to optically detonate binary explosive mixtures of concentrated nitric acid and organic fuels as it was discovered that these liquids are far more difficult to detonate than reported in the literature. This difference is likely due to the liquid mixtures deflagrating when exposed to external stimuli, consistent with the safety warnings about working with these materials but failing to detonate due to the limited shock heating experiences by liquid explosives due to their lack of inhomogeneity.
The unexpected stubborn reluctance of nitric acid to detonate led the team to test whether a convention secondary explosive (PETN) could be detonated with the shocked xenon light source. The shocked xenon light source was able to heat the PETN to the point where it was detonated by the arrival of the weak from the xenon light source at the target plane but was unable to achieve optical detonation. In partnership with Dr. Ben Fuller at Battelle, the team was able to test the optical initiation of DBX-1 (a green primary explosive being developed by the Army Research Laboratory) using the optimized shocked xenon light source. The charge of DBX-1 clearly detonated from the incoherent radiation from the xenon light source, confirming the feasibility of such a system.
Conclusions and Path Forward
This project has demonstrated that explosively shocked xenon light sources are capable of outputting tremendous amounts of visible and ultraviolet light from a relatively simple design. This project has also demonstrated that liquid mixture of nitric acid and organic fuels, while susceptible to deflagration in the presence of weak external stimuli, are not easily detonated when subjected to intense radiation of explosive shock. Through collaboration, the team has demonstrated that our explosively driven xenon light source can be used to optically detonate primary explosives (DBX-1) but could not quite cross the necessary threshold to detonate secondary explosives (PETN). While the success with DBX-1 is promising, the required contact operations with a primary explosive precludes further development at the NNSS, but additional follow-on funding is being pursued by our collaborators who have the proper facilities and knowledge to pursue primary explosives research. However, the explosive containment vessels and the safety basis for performing high hazard small-scale explosives operations at the Special Technologies Laboratory have been integrated into a variety of other NA-10, NA-20 and SDRD-funded projects, enabling a host of new experiments relevant to the NNSS mission.
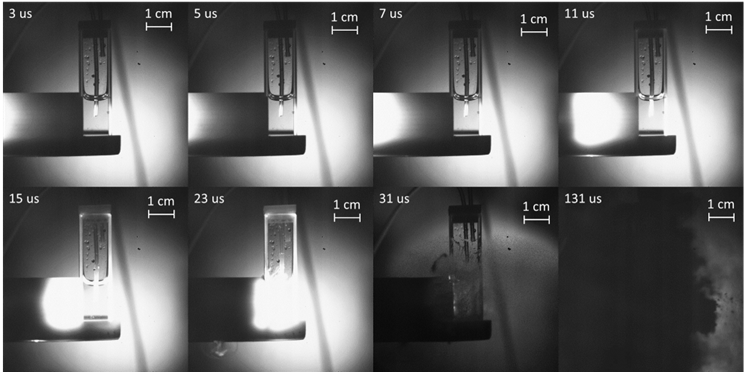