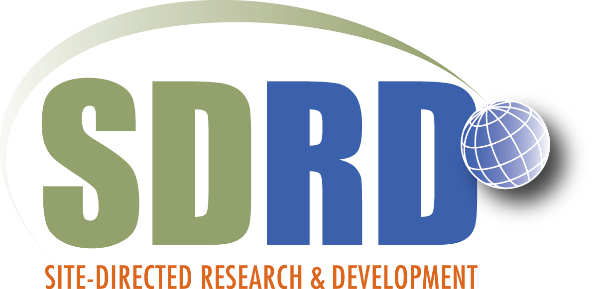
Measurement of Dynamic Melting and Re-Crystallization of Shocked Metals
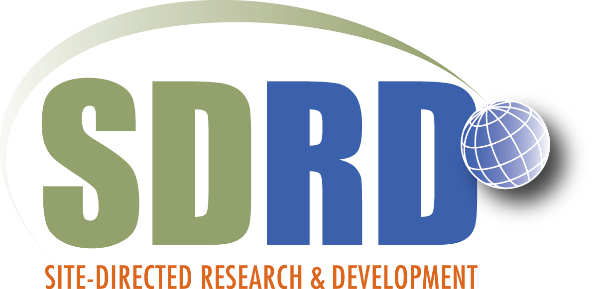
Project # 23-060 | Year 1 of 3
R. Jason Scharffa, Brandon La Loneb, Gerald Stevensb, Matt Staskab, Ruben Valenciab, William Turleyb
aLos Alamos Operations (LAO), bSpecial Technologies Lab (STL)
This work was done by Mission Support and Test Services, LLC, under Contract No. DE-NA0003624 with the U.S. Department of Energy, the NNSA Office of Defense Programs, and supported by the Site-Directed Research and Development Program. DOE/NV/03624–1891.
Abstract
Shock-induced phase transformations are of seminal importance to the weapons physics community. The dynamic trajectory of a nuclear primary prior to maximum compression is quite complex. Materials in the primary are subjected to complex loading conditions composed from multiple shock compression waves, rarefaction waves, and ramp compression waves. As a result, those materials will traverse a range phase space that can induce solid-solid and solid-liquid phase transformations. This project is exploring the use of velocimetry, radiance, and reflectance techniques to measure time‐resolved pressure-temperature pathways of a shock‐compressed metal. This was done to support recent and future pyrometry experiments for measuring the dynamic melting and re-crystallization of shocked metals.
Background
Melting and re-crystallization of shocked materials is very difficult to detect using the typical shock wave diagnostic of velocimetry because density changes at these boundaries are small. Advances in x-ray diffraction have shown that re-crystallization can be observed dynamically; however, these measurements are typically performed at second or third generation light sources at user facilities such as the Advanced Photon Source at Argonne National Laboratory. Moreover, x-ray diffraction is not yet able to measure where in temperature-pressure space the re-crystallization occurs in comparison with the equilibrium phase boundary. The direct measurement of shock melting and re-crystallization has generally been a longstanding problem in the dynamic compression community, and particularly so with respect to nuclear primary physics. Our technique can be rapidly adapted to TA-55 and Joint Actinide Shock Physics Experimental Research (JASPER) for Special Nuclear Material (SNM) measurements and provides accurate and time resolved temperature and pressure data for determining if and where the re-solidification occurs. This will more than likely be the preferred tool directly measuring the phase behavior of SNM at TA-55, JASPER, and U1a.
Technical Approach
The primary objective of this work is to investigate the recompression pressure‐temperature pathway of shock melted metals to determine where re‐solidification occurs in comparison with the equilibrium phase boundary. Shock‐release‐isentropic recompression plate impact experiments were conducted at the Special Technologies Laboratory (STL) and diagnosed with the STL‐developed phase and temperature diagnostic using simultaneous radiance and reflectance spectroscopy. We chose to study the melt and re‐crystallization in tin (Fiscal Year (FY) 2021 and FY 2022) because it has (1) a phase diagram with characteristics of interest to the weapons program, (2) a relatively high atomic number, and (3) the relevant pressure‐density states are accessible to our guns located at STL. For the third year (FY 2023) of this project, we developed a pre-heated target assembly where the tin target is shock loaded from the liquid phase entirely. For emphasis, the basic formula of a general equation of state (EOS) is related to three thermodynamic variables of pressure (P), temperature (T), and volume (V), which are macroscopically measurable parameters for describing the physical state of matter and form the starting point for all calculations of weapons physics. To examine the validity of theoretical models of the EOS on the principal Hugoniot, shock experimental data are required not only for the thermodynamic variables of pressure and volume, but also for temperature.
Results and Technical Accomplishments
In FY 2021 and FY 2022, we mapped the time-dependent pressure-temperature phase space of tin that has been shock loaded, released, and subsequently recompressed using the STL powder gun. Details of the target design have been reported in Site-Directed Research and Development quarterly reviews and are briefly summarized here. The experimental configuration consists of a 1 mm tin sample separated by a 100‑micron vacuum layer to a thin layer of PMMA (low impedance transparent solid) which is backed by a lithium fluoride anvil (high impedance transparent solid). The impactor is composed of tin. The impactor is accelerated by the STL powder gun to a velocity of 2.5 km/s at impact. The tin sample is initially shocked to a high-pressure solid state followed by a full release to zero stress when the shock wave reaches the free-surface at the back of the target about 50 ns after first shock. The tin sample is partially melted when recompression begins. Re-solidification is accomplished through a series of wave reverberations within the low impedance layer to recompress the tin sample along a quasi-isentropic path. Finally, the fully re-solidified tin sample reaches a peak stress approximately 250 ns after recompression. From the P-T shape of the recompression path, we have determined where re-solidification in tin occurs relative to the equilibrium solid-liquid phase boundary. However, because we are only looking at the back surface of the tin target, we cannot claim that the target was completely melted prior to re-solidification.
In FY 2023, the new sample preheat capability allowed us to shock compress the tin sample directly from the liquid phase. The sample is shocked to 14 GPa along the liquid Hugoniot where it reaches a steady pressure state, then isobarically heats up towards the equilibrium phase boundary.
Conclusions and Path Forward
We have determined that in a multi-phase metal, such as tin, the initial conditions of the sample matter to the thermodynamic pathway the material takes under dynamic loading conditions. Our phase/temperature diagnostic shows that the phase change kinetic pathway depends on if the metal is partially or fully melted.
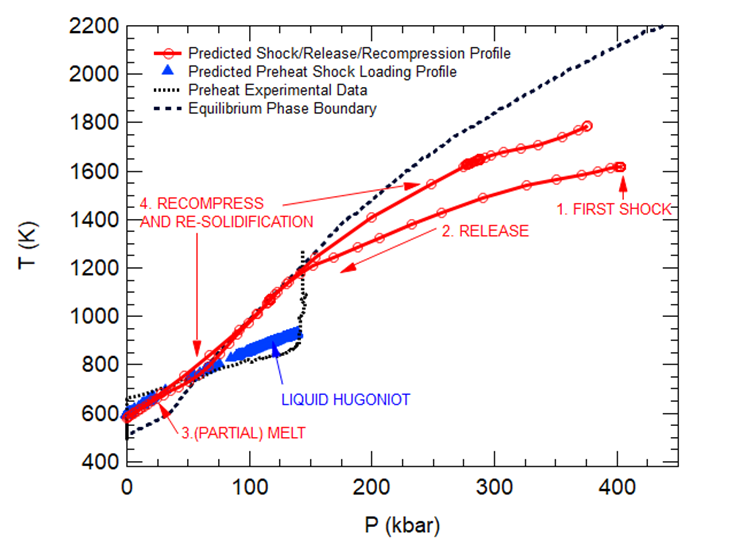
Publications
- Title: Hyperfine structure and isotope shifts of xenon measured for near-infrared transitions with Doppler-free saturated absorption spectroscopy
Journal / Conference: Spectrochimica Acta Part B: Atomic Spectroscopy 202 (2023) 106635
Year: 2023
Author(s): James Bounds, Alexandre Kolomenskii, Rusty Trainham, Manuel Manard, Hans Schuessler