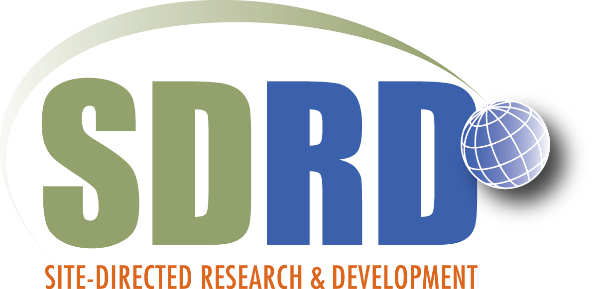
Spatial Spectral Observations from Near and Far
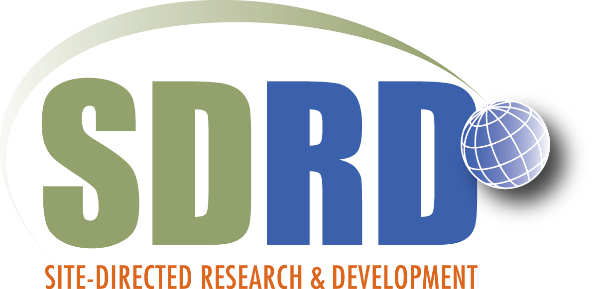
Project # 23-095 | Year 1 of 3
Michael Howard
Remote Sensing Lab – Nellis (RSLN)
This work was done by Mission Support and Test Services, LLC, under Contract No. DE-NA0003624 with the U.S. Department of Energy, the NNSA Office of Defense Programs, and supported by the Site-Directed Research and Development Program. DOE/NV/03624–1906.
Abstract
The aim of this project is to identify spatial and spectral signatures of surface materials that characterize the experimental design and proliferation intention of underground weapons testing in vertical emplacement shafts. Early detection and characterization of an underground weapons testing program is critical intelligence for the formation of national nuclear proliferation policy but is subject to denied access and intentional concealment to conventional detection methods making optical remote sensing an ideal tool allowing remote detection and surveillance capabilities of underground construction activities. While underground, there are many surficial signatures surrounding the construction and use of subsurface testing grounds that will be investigated from a spatial-spectral perspective. This research will collect coordinated airborne imaging and ground-based spectral measurements of the Rock Valley Direct Comparison (RVDC) site during its construction, testing, and shutdown and analyze the data using spatial-spectral convolution techniques to simulate current and in-development satellite-based and high-altitude aircraft-based surveillance optical imaging systems.
Background
The early detection and characterization of underground weapons testing programs is critical intelligence for the formation of national nuclear proliferation policy but is subject to denied access and intentional concealment to conventional detection methods. Detection of nuclear testing by nefarious state actors is made difficult due to their concealment efforts primarily by testing underground to limit surface signatures. Therefore, well vetted detection methods like the global seismic network measurements have been developed that record seismic signals and can detect underground weapons testing events. However, the size and magnitude of the weapons explosive is very difficult to quantify without knowing the depth of burial of the explosive device and the geologic properties surrounding the explosive. Being able to quantify the event is very important if an accurate device yield estimation is to be calculated. An estimate of the device yield is critical information in confirming an adversarial nuclear weapons program capability. However, even subsurface testing can generate many surficial signatures during construction and weapons testing that can be exploited from a spatial-spectral perspective with optical remote sensing technologies. Optical remote sensing is an ideal tool that allows remote detection and surveillance capabilities that can overcome access limitations and provide long-distance detection and characterization. Considering the nature of remote sensing, optical remote sensing methods will be researched to identify spatial and spectral signatures of surface materials that characterize the experimental design and proliferation intention. This will expand the application of optical systems (hyperspectral and broadband) to obtaining technical intelligence on vertical underground weapons testing observables and corroborating evidence of testbed depth and geologic content. Depth of burial estimates and geologic characterization of the testbed determined through remote sensing methods can then be provided to the seismic community to calculate a better yield estimate.
Technical Approach
Using the RVDC testbed, key observables will be examined to test optical dependencies from multiple data collection modalities including visible to shortwave infrared imaging and single pixel spectrometers (in situ) offering varying spectral resolution and ranges coupled with varying ground sample distances. The data collections will be used to analyze the strengths and weaknesses of each modality. An airborne hyperspectral instrument is used to collect high resolution image data and will be flown at a high altitude to simulate satellite-based collections. Spectral convolution techniques are utilized to match other sensor spectral responses allowing these different modalities (spatial and spectral) to be equitably compared as they are collected in near time of each other.
The primary observable at the RVDC testbed will be centered on drilling activities. The act of drilling will provide both anthropogenic and geologic signatures. A central hypothesis for the geologic portion of this research is to interpret the spectral content of the cuttings to estimate depth of drilling. To ensure an accurate account of this relationship, spectral measurements, and metadata from Test Well F core near the RVDC site will be collected. Core taken from the exploratory borehole on the RVDC site at the device emplacement shaft will confirm the relationship of drill depth versus mineral classification and will be used as a baseline for the airborne measurements.
Anthropogenic activity will generate signatures that indicate drilling intent. From a nuclear testing perspective, actions that greatly dictate design and intent include containment of radionuclides, treaty compliance, health and safety guidelines, and concealment for undeclared testing. The two categories (small and large explosions) of testing events have the same general requirements but differ in magnitude of operation. The phases that form the event site are introduced by anthropogenic activity and are comprised of:
- Site selection and drill operations
- Site construction including concrete work and buildup for other test infrastructure
- Device delivery and assembly
- Diagnostic preparation and assembly
- Down-hole emplacement of the experiment
- Test execution
- Post-shot operations
Many of the anthropogenic signatures will be observed in the spatial domain of optical measurements. The spatial domain can only be useful if the ground sample distance (GSD) is sufficient to resolve spatial shape of relevant drilling indicators to distinguish testing intent. After key indicators are determined, a suitable GSD will be verified by assessing different collection geometries. The geologic and anthropogenic signatures and other spectral and spatial discoveries will be evaluated by analysis of the data collected at varying collection modalities. This analysis will involve calibrations of visual observations using ground truth data and logs and assessed with a high-resolution true color orthorectified image mosaic and digital surface model of the RVDC testbed. This visual data will be collected concurrently with hyperspectral airborne collections and can be used as a referee product for the hyperspectral collection modalities as well as an accurate base map for all RVDC researcher needs.
Results and Technical Accomplishments
The primary research objective and confirmation of the theoretical framework of this project was establishing the relationship between spectral content of drill cuttings and core to estimate depth of drilling. The initial findings were confirmed using spectral measurements and metadata from Test Well F (TW-F) cuttings and core that were derived from the US Geological Society Core library. Very Near Infrared (VNIR)/Short Wave Infrared (SWIR) and Long Wave Infrared (LWIR) spectra were collected from ~175 depths of TW-F core/cuttings ranging from 30 to 3,400 feet along with metadata that included x-ray fluorescence (elemental composition), context photos, and macro photos. In addition, 173 samples were taken from the same locations and analyzed by x-ray diffraction analysis at New Mexico Tech to determine complex mineral formula. The attached graphic illustrates a colorized spectral waterfall plot and shows that the lithologic changes with depth are spectrally separable. Additional analysis using the TW-F spectral data included mineral and rock type classification using both multivariate and matched filter analysis software tools. For anthropogenic activity, both spatial and spectral domain observables were measured in the first year using airborne data that collected both background and first ground break construction. From a spatial perspective, the drill pad construction at the RVDC site obviously produced the first indication of a future drilling activity. Ortho-mosaic data provided precise location, size, and terrain elevation of the drill pad that could determine the grading extent and material disposition and the VNIR/SWIR data was used to spectrally separate the new drill pads from existing ones within the vicinity.
Conclusions and Path Forward
The first year of this research was highly successful as three primary objectives were completed. Both anthropogenic and geologic observables and signatures were discovered in the first year of this research. Moving forward in the second year we will continue the airborne data collections of the RVDC site to establish key observable and test the optical dependencies. In addition, we will develop the convolution strategies for multiple modalities and continue rock and mineral classification using the two analytical approaches mentioned above.
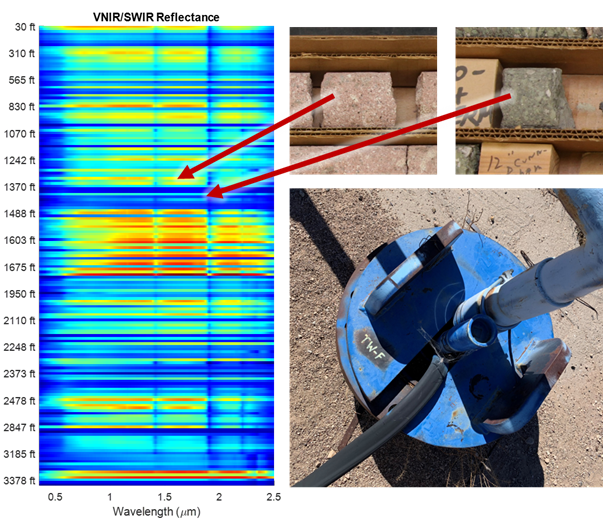