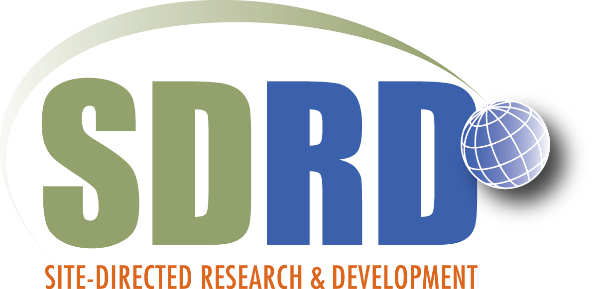
Micro‐Ion Traps for Real‐Time Chemical Analysis in Harsh Environments
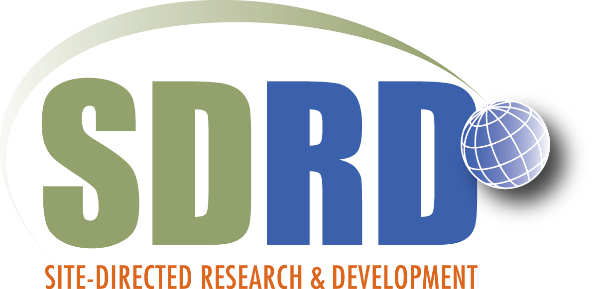
Project #: 22-003 | Year 3 of 3
Manuel J. Manard,a Rusty Trainham,a Paul Kemperbb
aSpecial Technologies Laboratory; bUniversity of California, Santa Barbara
Executive Summary
The goal of this project is to design, build, and test a proof-of-concept prototype utilizing small Paul-type (radio-frequency (RF) field) ion traps. These will provide mass spectral analysis of chemical species, which will be used specifically for making measurements remotely in harsh environments.
Description
This effort seeks to design, build and test proof-of-concept portable mass spectrometer using Paul-type ion traps, having trapping regions measuring hundreds of microns, to provide real-time chemical analysis in the field. The unit is being designed for remote operation, such as on unmanned aircraft system (UAS) platforms. The goal is to meet the weight and power operational requirements of small quadcopter platforms that have been used as part of several previous UAS chemical sensing tests. Specifically, a maximum weight of 750 g and power draw less than 3 W is the objective. We will also attempt to exceed the sensitivity of the nonspecific, real-time chemical sensors previously flown on UASs and thus, a sensitivity of less than 1 ppm is targeted.
At the completion of Year 2, data were acquired demonstrating ion confinement in the micro-trap (z0 = 250 µm, defined as the distance from the center of the trap to tip of an end cap). These data were acquired with an RF frequency of 25 MHz and amplitude of 40 Vp. After achieving ion confinement, data were acquired illustrating mass-selective ejection from the trap. Here, a second RF frequency was applied to one end cap electrode. The second RF frequency is used to drive ion motion inside the trap, destabilizing ion trajectories and causing ejection from the trap. The specific frequency leading to ejection from the trap is inversely proportional to the mass-to-charge ratio (m/z) of the confined ion. By scanning the drive frequency (Ωd), mass analysis of ions in the trap is obtained.
Using results obtained in Years 1 and 2, the resolution for mass-selective ejection from the micro-ion trap was significantly improved in Year 3. These data were acquired by applying Ωd to the trap while ions were confined inside and then using the negative voltage pulse to probe the trap for the presence of ions within. If Ωd forced ions out of the trap, no signal would be measured when the pulse voltage was applied. Ωd was then stepped and the process repeated for a range of frequencies. Optimization of experimental parameter applied to the trap led to a measured full width, half maximum (FWHM) resolution of approximately 54 (fig. 1a). This is a seven-fold increase in the FWHM resolution of the system when comparted to data acquired in Year 2. Ion confinement and mass-selective ejection using Xe+ was also demonstrated during Year 3 (fig. 1b). Here, three features were observed in the peak corresponding to Xe+, consistent with the multiple naturally-occurring isotopes of Xe, ranging from m/z = 124 to 136. These three features, along with the peak observed for mass-selective ejection of Ar+ were used to generate a plot of m/z versus (1/ Ωd2)) having an R2 = 0.991. The high degree of linearity of these data are a strong indication the ion trap in functioning correctly.
Finally, a printed circuit board (PCB) was designed that contains an array of nine micro-ion traps (fig. 1c, traps are labeled Tr1–Tr9 and are shown in yellow). The PCB is 1″ in diameter and 0.125″ thick. The array will mitigate the reduction in sensitivity resulting from the reduced size of the trap caused by Coulomb repulsion (smaller traps necessarily hold fewer ions). This simplifies the design of the system, leading to a device that could potentially be produced quickly and inexpensively.
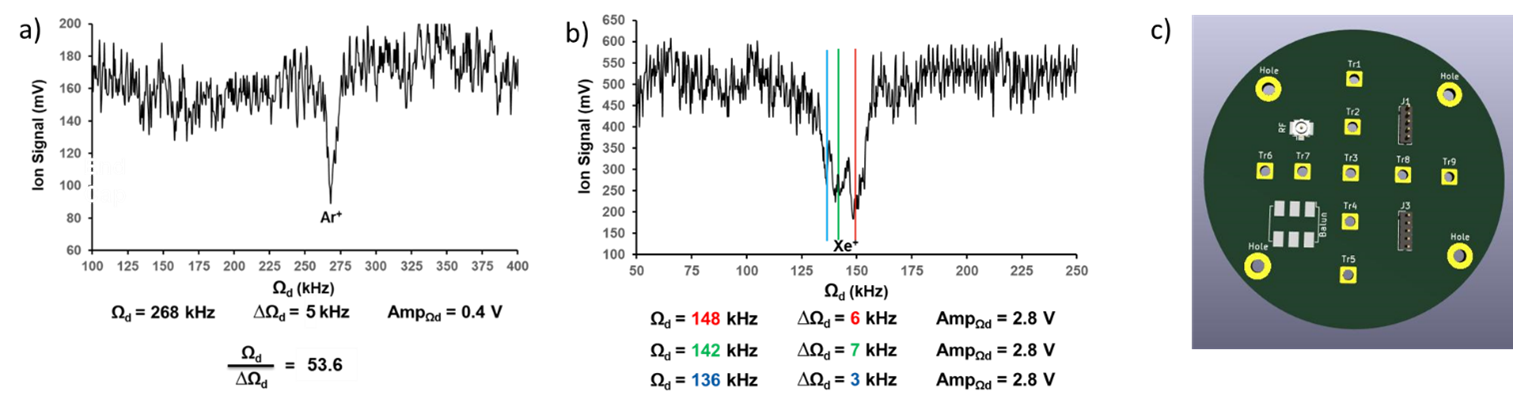
Conclusion
The performance of the micro-ion trap, with z0 = 250 µm, was evaluated and improved during Year 3. Data were obtained demonstrating significant improvements in mass resolution, extension of the trap’s mass range and isotopic analysis using Xe. A PCB measuring 1 inch in diameter and 0.125 inches thick has been designed containing an array of nine micro-ion traps.
Mission Benefit
The development of small, field-portable systems for chemical detection and identification is of interest to multiple government agencies. Inexpensive instruments that are capable of providing real-time chemical analysis with reasonable sensitivity and selectivity in remote and/or harsh environments, such as on a UAS, is desired. A device capable of providing analytical specificity in the field and transmitting the data back to the operator would be a significant improvement for certain operational scenarios.
Publications, Technology Abstracts, Presentations/Posters
A publication detailing the design and performance of the micro-ion trap is in preparation for submission to a peer-reviewed journal.
This work was done by Mission Support and Test Services, LLC, under Contract No. DE-NA0003624 with the U.S. Department of Energy. DOE/NV/03624–1628.
Return to Enabling Technologies for Autonomous Systems and Sensing
Go to SDRD Annual Report Index
Return to SDRD Homepage