Behind the scenes of every subcritical experiment (SCE) executed at the Nevada National Security Site (NNSS) is years of collaborative development between scientists at the NNSS and NNSA National Laboratories.
Components of comprehensive SCEs are developed and produced at various centers of diagnostic expertise. To name a few, shock-wave diagnostics and instrumentation development take place at the NNSS’ Special Technologies Laboratory (STL) in Santa Barbara, California; calibration for optical, X-ray and laser systems occur at the NNSS’ Livermore Operations in Livermore, California; electronic imaging, optical systems and instrumentation happen at the NNSS’ Los Alamos Operations in Los Alamos, New Mexico; and electro-optics, fiber and control systems are provided at the NNSS’ Nevada Operations in North Las Vegas, Nevada.
SCEs performed at the NNSS’ U1a Complex, the underground laboratory used to acquire data for the NNSA’s Stockpile Stewardship Program, derived from the 1992 nuclear testing moratorium.
“For every SCE that we execute, each series is a significant hurdle,” said NNSS Detectors and Instruments Project Manager Amy Lewis. “Diagnostics are imagined to answer the questions posed by laboratory scientists. Our developers design the hardware to take the data to validate weapons models.”
The process can take years. Lewis has served the NNSS for 21 years and has had the unique perspective of working onsite at the U1a Complex during SCE execution as well as supporting diagnostic development in New Mexico. Though SCE experiment development can take a long time in the context of one’s career, the historical progression is colossal.
“When we think about where we are in weapons experiments, the low-hanging fruit is done,” said Lewis. “Stockpile Stewardship drove diagnostic development precision from the 10 percent regime to the one percent. It’s not unusual for experimental capabilities to take 10 years for development.”
As reference to how far imaging alone has come in several decades, Lewis, whose current work focuses on a new field camera prototype, points to Harold Edgerton’s famous 1957 “Milk Drop Coronet,” a stop-motion photograph capturing the moment a drop of milk makes contact with a table. Edgerton’s work was a landmark moment in photography, demonstrating how stills can impact human understanding of physical events.
“Edgerton led the diagnostic conception of this contract in the 1950s, and his mark is still evident in our contribution,” said Lewis. “Diagnostic imaging and optical system development remains a core competence for us.”
In a career that’s a marathon, not a sprint, Lewis says their diagnostic data validates and sometimes contradicts current laboratory weapons models.
“When data doesn’t align with the physics model, that’s the real win,” said Lewis.
Diagnostic development
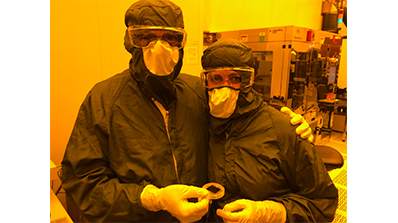
Senior Principal Scientist Jason Scharff, who has a shock and detonation physics background, came to the NNSS in 2017 after spending 11 years at Los Alamos National Laboratory (LANL). His first work with the Site began with solving scientific technological problems related to the stockpile and primary physics, including developing optical velocimetry probes for Joint Actinide Shock Physics Experimental Research Facility experiments. As his tenure grew with LANL, he began to work with STL scientists who were developing temperature diagnostics, now his current work.
Scharff examines crystal plasticity, looking at the state and strength of a metal as it is compressed. He collaborates with LANL and Sandia National Laboratories to obtain experimental data for plasticity of single crystal materials.
“The mission, fundamentally, is very specialized,” said Scharff. “Weapons designers reply on inputs to code to simulate the system. Inputs to those models are state of material, pressure, volume and temperature.”
Now in the research phase, his team will design experiments that diagnose the physics of plastic deformation. The diagnostic is then brought to the laboratory, which may design a subcritical experiment around it.
“It takes a lot of trial and experiment,” said Scharff. “There are a lot of bits and pieces that go into that development—a lot of development. The labs will come to us and say we want to do x, y and z, and we have teams that do those things.”
Fueling the future
Lewis and Scharff also play roles in introducing the NNSS to newer faces, from first-year summer interns to post-doctoral researchers.
“We certainly need early-career folks to bring energy and bravery to solving the tech problems we face,” said Lewis. “I’m excited that the early-career workforce challenges us to explain the broader perspective. The next generation will solve problems with a more top-down perspective.”
Diagnostic development continues to advance not only through enhanced technological capabilities, but also to meet the demands posed by evolving national security challenges.
“Having worked in multiple locations, I have a unique perspective when I’m planning diagnostics,” said Lewis. “I understand the environment where the equipment has to perform. Diagnostics of the future are going to adapt to data streams while employing telecom-imagined user interfaces and control methodologies. The world’s hazards are rapidly changing; our core competencies will help us adapt to those changes while acquiring better data for our sponsors and colleagues.”